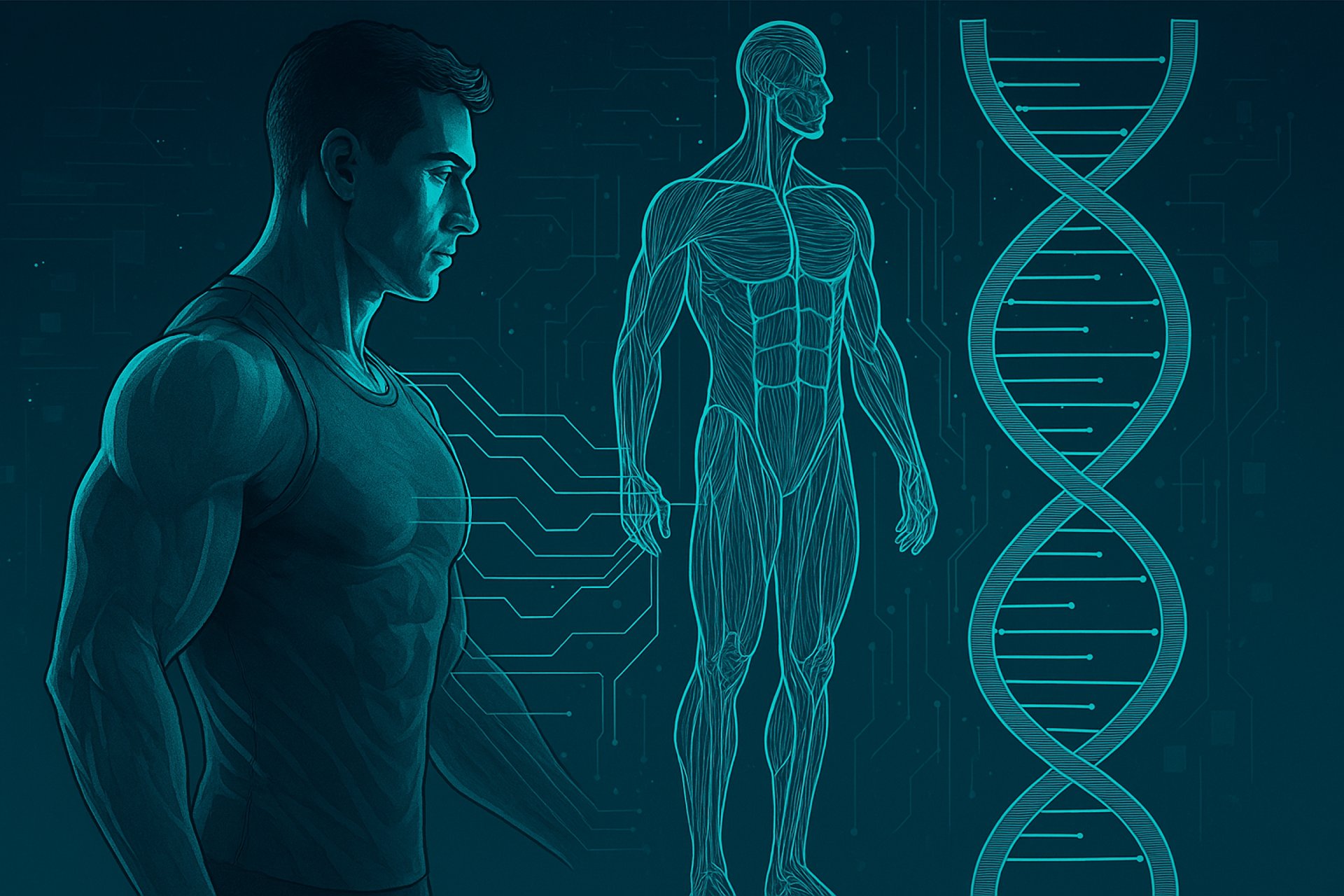
The Future of Fitness
Synthetic Biology & Muscle Enhancement
Why Synthetic Biology Is the Next Frontier in Muscle Enhancement
We are entering an era where the boundaries between biology, technology, and athletic performance are blurring at an unprecedented pace. While traditional fitness methods—like strength training, nutrition, and supplementation—still form the bedrock of physical development, the future promises an extraordinary upgrade: synthetic biology muscle enhancement.
This field merges genetic engineering, biotechnology, and molecular programming to rewire the way our muscles grow, repair, and function. From CRISPR gene editing to lab-grown muscle tissue, what once sounded like the realm of elite science is now within striking distance of real-world application—especially in the realm of sports, recovery, and physical longevity.
But with innovation comes controversy. What are the ethics of engineered muscles? Who gets access to these enhancements? And could this spark a new divide between the biologically enhanced and the naturally trained?
In this deep dive, we’ll explore what synthetic biology is, how it’s being used to enhance muscle function, where the fitness industry is heading, and what it means for athletes, weekend warriors, and fitness enthusiasts alike.
What Is Synthetic Biology?
Synthetic biology is a cutting-edge scientific discipline that involves redesigning organisms by engineering them at the molecular and genetic level. Unlike traditional genetic modification—which typically involves inserting genes from one organism into another—synthetic biology goes a step further. It allows scientists to construct entirely new biological parts, systems, and even organisms from scratch, using principles borrowed from computer science, engineering, and biotechnology.
At its core, synthetic biology aims to make biology programmable—like software. Just as engineers write code to create apps, synthetic biologists write genetic “code” (sequences of DNA) to program how cells behave. These custom instructions can tell a cell to produce a specific protein, respond to environmental changes, or self-destruct when no longer needed.
Key Features of Synthetic Biology:
Modular Design: Genes, proteins, and other biological elements are treated like building blocks that can be mixed, matched, and assembled to create new biological systems.
Standardization: Genetic elements can be designed using standardized parts, often cataloged in open-source repositories like the BioBricks registry.
Automation & AI: Machine learning and automation tools are now integrated into the design and testing process, accelerating discovery and reducing trial and error.
Predictable Outputs: By using computer models to simulate how a gene circuit will behave, researchers can predict and fine-tune the results before actual testing.
How It Differs from Traditional Biotech
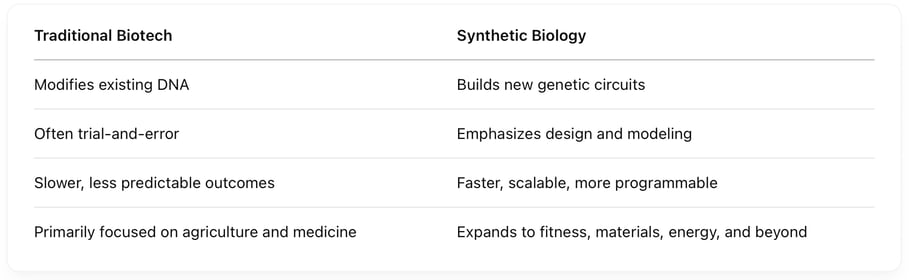
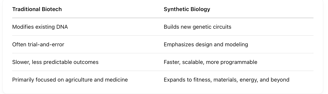
Applications Relevant to Fitness
In the realm of fitness and human performance, synthetic biology is being used to:
Engineer muscle cells that grow faster or repair more efficiently
Suppress genes that limit hypertrophy (e.g., myostatin inhibition)
Enhance energy production by optimizing mitochondrial function
Create synthetic proteins that outperform their natural counterparts
This level of control opens the door to muscle enhancement not just through training and nutrition—but through cellular engineering, potentially rewriting the rules of physical performance.
Muscle Enhancement 2.0: New Frontiers in Human Performance
We’re entering a new biological era—where muscle performance can be engineered, not just trained. Synthetic biology is laying the foundation for next-level muscle enhancement, pushing far beyond protein powders and resistance training.
CRISPR and Gene Editing for Muscle Growth
CRISPR-Cas9 is the revolutionary gene-editing technology that allows for ultra-precise modifications to DNA. It works like molecular scissors—targeting specific genetic sequences, cutting them, and allowing for the insertion, deletion, or replacement of genetic material.
In the context of muscle enhancement, CRISPR opens the door to rewriting how our muscles grow, repair, and recover. In animal studies, CRISPR has been used to:
Knock out the myostatin gene: Myostatin is a natural protein that limits muscle growth. Inhibiting this gene leads to significantly larger muscle mass—seen in breeds like the Belgian Blue cattle and myostatin-deficient mice.
Enhance IGF-1 (Insulin-like Growth Factor 1) pathways: IGF-1 plays a central role in muscle repair and hypertrophy. Editing genes to increase IGF-1 expression could promote faster recovery and greater growth after workouts.
Combat muscle atrophy: By targeting genes associated with sarcopenia (age-related muscle wasting) or cachexia (muscle loss from chronic illness), CRISPR has been shown to reduce catabolic markers in aging animal models.
Human Potential
While the use of CRISPR for muscle enhancement in humans is still in the research or experimental biohacking stage, the implications are enormous. Theoretically, a single genetic intervention could provide permanent or semi-permanent benefits in muscle mass and performance.
Potential future applications may include:
One-time genetic “boosts” for athletes or soldiers
Preemptive gene therapy for those at risk of degenerative muscle disease
Personalized genetic training optimization—where your genes determine your ideal workout style
⚠️ Important Note: Human gene editing for enhancement purposes raises significant ethical, legal, and safety issues. While therapeutic CRISPR trials are underway for rare diseases, enhancement applications are not yet approved, and long-term effects remain unknown.
Synthetic Myocytes: Lab-Grown Muscle Tissue
The development of synthetic or engineered muscle tissue—often called lab-grown or in vitro myocytes—is advancing rapidly in biomedical research. These are muscle cells created in a lab setting using stem cells, genetic programming, or tissue scaffolding technologies.
These myocytes can:
Contract spontaneously
Respond to electrical or chemical stimuli
Fuse with existing muscle fibers once implanted
Real-World Applications
This technology is already being investigated for treatment of traumatic injuries, such as in military or burn victims, but it holds potential far beyond medicine.
In a fitness and performance context, lab-grown muscle cells could be:
Injected into underperforming or damaged muscle groups
Programmed for enhanced oxygen use, recovery speed, or metabolic efficiency
Used to “upgrade” muscle density or fiber type on demand
Imagine targeted injections to restore muscle mass post-injury or even enhance your quads before a competition.
Regenerative and Modular Potential
This could lead to modular muscle enhancement—where athletes selectively enhance specific muscle groups for strength, speed, or endurance. Coupled with neural integration, synthetic muscle fibers might even outperform natural ones in durability and fatigue resistance.
This approach would fundamentally transform rehabilitation protocols, potentially shortening recovery time by months and preserving functionality in severe trauma cases.
Genetic Implants & Programmable Proteins
Another exciting frontier is the development of genetic implants and programmable proteins—synthetic biological tools designed to fine-tune muscle performance at the cellular level.
These enhancements might come in the form of:
Programmable Protein Devices
Synthetic actin and myosin analogs: These are custom-designed muscle fibers engineered for stronger, more efficient contractions. Unlike natural proteins, these can be optimized for performance or longevity.
Artificial mitochondria: Enhanced cellular power plants designed to generate more ATP (cellular energy), potentially delaying fatigue during intense workouts or endurance events.
Protein synthesis regulators: Bioengineered agents that activate anabolic pathways like mTOR on demand, triggering muscle growth even without the same level of training stimulus.
Smart Implants with Real-Time Control
Startups are already working on wearable or implantable devices that deliver genetic or protein-based “boosters” in real time. These might use:
AI-integrated sensors to monitor muscular stress, hormone levels, or fatigue
App-controlled delivery systems that activate gene sequences or release performance molecules when needed
Closed-loop feedback systems that adjust enhancement protocols based on training data
This could mean you walk into a gym, and your wearable decides it’s time to increase protein synthesis in your legs—activating a gene sequence before your squats even begin.
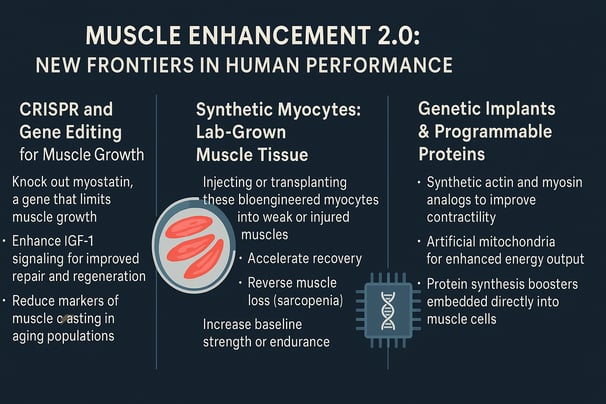
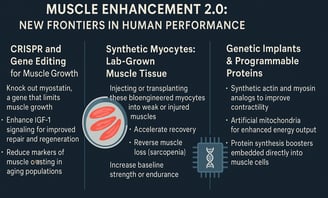
Ethical, Legal, and Social Concerns
The ability to reprogram our bodies at the genetic level—especially for performance purposes—raises profound ethical, legal, and social dilemmas. As synthetic biology and muscle enhancement technologies accelerate, the questions are no longer just “Can we?” but “Should we?” and “Who gets to decide?”
Where Do We Draw the Line?
Gene doping—the use of genetic engineering to improve athletic performance—is already banned by the World Anti-Doping Agency (WADA). But the distinction between medical therapy and performance enhancement can become blurry. For example, using gene therapy to restore muscle function after injury is allowed, but using the same therapy to increase muscle mass beyond natural limits is not.
Key concerns include:
Detection problems: Gene edits are internal, often indistinguishable from natural genetic variations. Traditional anti-doping tests can't easily identify them.
Enforcement gaps: Global regulation of gene editing in sports is inconsistent. Some countries may ban it outright, while others may allow certain applications.
Definition of fairness: If some athletes are genetically enhanced and others are not, do traditional notions of "level playing field" still apply?
As technology progresses, sports may be forced to divide into “natural” and “enhanced” leagues, much like current debates around gender categories and technological aids (e.g., carbon fiber prosthetics in sprinting).
Equity and Access
Cutting-edge biotech is expensive—and this raises serious questions about who benefits from it.
Will elite athletes and the ultra-wealthy gain early access to enhancement therapies, creating a biological class divide in sports and fitness?
Could this trigger a new wave of “performance inequality,” where access to synthetic muscle enhancement becomes the modern equivalent of exclusive training facilities or banned substances?
Will global disparities worsen, with developing nations falling further behind in athletic competition and health technologies?
Without equitable access and regulation, synthetic biology may reinforce existing systemic inequalities in both healthcare and competitive arenas.
Consent, Autonomy & Safety
Gene editing carries unpredictable risks, especially when applied to healthy individuals for non-medical reasons. Some key concerns include:
Off-target effects: CRISPR can accidentally edit unintended sections of DNA, potentially triggering cancer or other genetic diseases.
Immune reactions: The body may attack edited cells or viral vectors used for gene delivery, leading to serious side effects.
Long-term effects unknown: We don’t yet understand how synthetic genes behave over years or decades. Changes might pass to future generations if done in germline cells.
Consent becomes even more ethically charged when considering minors, elite athletes under pressure to perform, or military personnel who may be asked to undergo enhancements for combat readiness.
Applications of Synthetic Biology in Fitness & Medicine
While much of the excitement around synthetic biology centers on performance enhancement, its most impactful applications may lie in rehabilitation, prevention, and long-term muscle health.
Athletic Applications
Faster Injury Recovery
Synthetic biology can regenerate muscle, tendon, and ligament tissues far more efficiently than traditional physical therapy alone. Engineered cells can be programmed to rapidly rebuild tissue at the site of trauma.Fatigue Resistance
By boosting mitochondrial efficiency or creating artificial mitochondria, cells can produce ATP more efficiently, delaying muscle fatigue and increasing endurance.Precision Nutrition Integration
Engineered muscle cells could be designed to respond directly to specific nutrients, enhancing amino acid uptake or regulating glucose use for optimal performance.Metabolic Reprogramming
Synthetic tools can influence how muscle fibers develop—encouraging a shift from slow-twitch endurance fibersto fast-twitch power fibers, or vice versa, depending on athletic goals.
Medical Applications
Sarcopenia and Muscle Wasting
Synthetic biology offers potential gene therapies or protein infusions to halt or reverse age-related muscle loss—potentially keeping seniors active and independent for longer.Muscular Dystrophy Treatments
Several types of muscular dystrophy, including Duchenne’s, are caused by single-gene defects. CRISPR and synthetic gene circuits could repair or replace faulty genes, offering new hope to those with inherited muscle diseases.Improved Diabetes Management
Muscles engineered to increase insulin sensitivity could help regulate blood sugar in diabetics—possibly reducing or eliminating the need for insulin injections in Type 2 diabetes.Post-Surgery and Bed Rest Recovery
Patients immobilized after surgery or injury could receive targeted injections of growth-inducing synthetic cells, reducing recovery time and preventing muscle atrophy.
In all these cases, synthetic biology may not just restore function—but enhance it beyond the patient’s pre-injury baseline.
The Rise of DIY Biohackers: Self-Experimentation in Muscle Enhancement
In parallel with academic research and regulated trials, a global subculture of DIY biohackers is experimenting with synthetic biology tools in garages, basements, and underground labs.
Driven by a desire to transcend human limits, these individuals blur the lines between curiosity, innovation, and risk. Notable trends include:
Common Practices
Injecting plasmids: Circular DNA structures programmed to boost gene expression (like muscle growth factors) are being synthesized and self-administered.
Using viral vectors: Similar to those in legitimate gene therapies, these viruses are loaded with genetic material and introduced to human cells—often without regulatory oversight.
Homemade CRISPR kits: Available online, these allow amateur users to attempt basic gene edits at home, though most are far from safe or effective.
High-Risk, High-Reward Culture
These biohackers often share results and methods in online forums and underground meetups. While a few claim noticeable muscle gain or enhanced recovery, outcomes are unpredictable, and the lack of clinical oversight is extremely dangerous.
Examples include:
Josiah Zayner, a well-known biohacker who publicly injected himself with CRISPR components to attempt gene editing
Amateur labs attempting to replicate myostatin gene suppression in humans, with little success and uncertain side effects
Implications
The DIY movement signals growing public demand for next-gen physical enhancement—especially from those who feel left behind by traditional fitness methods. It also challenges policymakers to create regulatory frameworks that protect the public without stifling innovation.
What Could Fitness Look Like in 2035?
Fast-forward to 2035, and stepping into a gym might feel more like entering a biotech clinic than a weight room. With synthetic biology muscle enhancement becoming mainstream, today's supplements and training plans could seem almost primitive by comparison.
Futuristic Fitness Scenarios
Genetically Tuned Training Plans
Your DNA is your new coach. Fitness platforms will analyze your genome to design hyper-personalized workouts, rest cycles, and recovery protocols based on how your body responds to stimuli at the molecular level.Muscle-Enhancing Vaccines
Need to maintain muscle mass during injury or aging? A single injection might activate anabolic genes or suppress muscle-wasting pathways for months at a time—no gym required.Smart Wearables That Repair You
Post-workout, your wearable detects microtears in your muscle and activates embedded proteins or genes to trigger instant repair responses—think real-time recovery.Subscription-Based Enhancement
Just like your protein powder delivery, you could subscribe to monthly gene infusions tailored to your goals—mass, endurance, speed, or all of the above.Biotech Gyms
These futuristic facilities combine traditional resistance training with on-site muscle therapy stations where clients receive performance boosters, metabolic recalibrations, or mitochondrial upgrades.
The New World of Competition
As performance enhancement becomes normalized, expect major shifts in how sports are structured:
Split Leagues: Natural vs. Engineered divisions in competitive sports and bodybuilding
New Standards: Genetic limits may be pushed so far that records and benchmarks must be reevaluated
Augmentation Regulations: Governing bodies will need to redefine what constitutes fair play in the age of programmable biology
Limitations and Challenges Ahead
While the vision is electrifying, several major hurdles could stall or reshape the rollout of synthetic biology in fitness.
Scientific Complexity
Muscle physiology isn’t controlled by one or two genes—it’s a network of thousands of interacting elements, including hormones, proteins, enzymes, and biomechanical forces. Editing one variable could cause ripple effects elsewhere.
Regulatory Bottlenecks
Gene therapies and synthetic biology applications will need approval from the FDA, EMA, and other regulatory bodies. The approval process for enhancements—not just therapies—will be long and politically charged.
Public Trust
Bio-enhancement is still viewed with skepticism. Fears of “unnatural” modifications, “Frankenstein fitness,” or genetic elitism could spark backlash and slow mainstream adoption—especially in conservative or risk-averse regions.
Biological Uncertainty
Even with the best planning, biology doesn’t always follow code. Risks include:
Autoimmune responses
Mutation errors
Unpredictable aging effects
Breakdown of synthetic proteins over time
The promise of better muscles could come with long-term trade-offs we’re only beginning to understand.
Final Thoughts: Should We Embrace Synthetic Muscle Enhancement?
The prospect of synthetic biology muscle enhancement is both thrilling and deeply polarizing. It promises to rewrite the limits of human performance, redefine the meaning of aging, and potentially erase diseases that have plagued muscles for centuries.
But that power comes with weighty questions:
Who decides what's enhancement and what's therapy?
How do we ensure equal access to these breakthroughs?
What happens to the culture of fitness when effort can be replaced—or amplified—by a shot of engineered code?
One thing is clear: the future of fitness will no longer be determined by training alone. It will be shaped by biology, code, and the values we bring to both.
For now, we’re standing at the edge of the next great performance frontier. And just like the strongest muscle fibers—it’s ready to flex.
References
National Human Genome Research Institute (NHGRI).
What is Synthetic Biology?
https://www.genome.gov/about-genomics/policy-issues/Synthetic-Biology#:~:text=Synthetic%20biology%20is%20a%20field,them%20to%20have%20new%20abilities.Nature Reviews Genetics.
Jinek, M. et al. (2012). A programmable dual-RNA–guided DNA endonuclease in adaptive bacterial immunity.
https://www.science.org/doi/10.1126/science.1225829World Anti-Doping Agency (WADA).
Gene Doping Overview.
https://www.wada-ama.org/en/resources/scientific-research/gene-doping-detection-next-generation-sequencingMIT Technology Review.
Regalado, A. (2018). First Human Embryos Edited in U.S.
https://www.technologyreview.com/2017/07/26/68093/first-human-embryos-edited-in-us/Cell Press: Trends in Biotechnology.
Zhang, J., & Williams, B. (2020). Engineering Muscles with Synthetic Biology.Science Advances.
Fischbach, M. A. & Segall-Shapiro, T. H. (2019). Building synthetic muscle: From bioengineering to augmentation.Harvard Medical School.
The Ethics of Human Enhancement: CRISPR and the Future of Bioengineering.Frontiers in Physiology.
Hoppeler, H. (2016). Molecular mechanisms of muscle plasticity with exercise and disuse.
https://pubmed.ncbi.nlm.nih.gov/23733647/Josiah Zayner – The Odin Biohacking Company.
Biohacking CRISPR Experimentation.
https://www.the-odin.com/diy-crispr-kit/NIH National Institute on Aging.
Sarcopenia and Age-Related Muscle Loss.
https://pmc.ncbi.nlm.nih.gov/articles/PMC6442923/